|
|
|
|
|
|
|
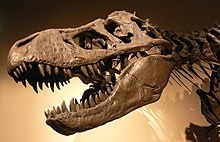 |
Tyrannosaurus rex. |
Paleontology
Paleontology, also spelled palaeontology or palæontology
(/ˌpeɪliɒnˈtɒlədʒi, ˌpæli-, -ən-/), is the scientific
study of life that existed prior to, and sometimes
including, the start of the Holocene Epoch (roughly
11,700 years before present). It includes the study of
fossils to classify organisms and study interactions
with each other and their environments (their
paleoecology). Paleontological observations have been
documented as far back as the 5th century BCE. The
science became established in the 18th century as a
result of Georges Cuvier's work on comparative anatomy,
and developed rapidly in the 19th century. The term
itself originates from Greek παλαιός, palaios, "old,
ancient", ὄν, on (gen. ontos), "being, creature", and
λόγος, logos, "speech, thought, study".
Paleontology lies on the border between biology and
geology, but differs from archaeology in that it
excludes the study of anatomically modern humans. It now
uses techniques drawn from a wide range of sciences,
including biochemistry, mathematics, and engineering.
Use of all these techniques has enabled paleontologists
to discover much of the evolutionary history of life,
almost all the way back to when Earth became capable of
supporting life, about 3.8 billion years ago. As
knowledge has increased, paleontology has developed
specialised sub-divisions, some of which focus on
different types of fossil organisms while others study
ecology and environmental history, such as ancient
climates. |
|
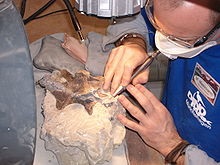 |
The preparation of
the fossilised bones of Europasaurus holgeri. |
Historical
science
William Whewell (1794–1866) classified paleontology as
one of the historical sciences, along with archaeology,
geology, astronomy, cosmology, philology and history
itself: paleontology aims to describe phenomena of the
past and to reconstruct their causes. Hence it has three
main elements: description of past phenomena; developing
a general theory about the causes of various types of
change; and applying those theories to specific facts.
When trying to explain the past, paleontologists and
other historical scientists often construct a set of one
or more hypotheses about the causes and then look for a
"smoking gun", a piece of evidence that strongly accords
with one hypothesis over any others. Sometimes
researchers discover a "smoking gun" by a fortunate
accident during other research. For example, the 1980
discovery by Luis and Walter Alvarez of iridium, a
mainly extraterrestrial metal, in the
Cretaceous–Tertiary boundary layer made asteroid impact
the most favored explanation for the Cretaceous–Paleogene
extinction event – although debate continues about the
contribution of volcanism.
A complementary approach to developing scientific
knowledge, experimental science, is often said to work
by conducting experiments to disprove hypotheses about
the workings and causes of natural phenomena. This
approach cannot prove a hypothesis, since some later
experiment may disprove it, but the accumulation of
failures to disprove is often compelling evidence in
favor. However, when confronted with totally unexpected
phenomena, such as the first evidence for invisible
radiation, experimental scientists often use the same
approach as historical scientists: construct a set of
hypotheses about the causes and then look for a "smoking
gun". |
|
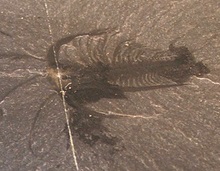 |
This Marrella
specimen illustrates how clear and detailed the
fossils from the Burgess Shale lagerstätte are. |
Sources of
evidence
Body fossils
Fossils of organisms' bodies are usually the most
informative type of evidence. The most common types are
wood, bones, and shells. Fossilisation is a rare event,
and most fossils are destroyed by erosion or
metamorphism before they can be observed. Hence the
fossil record is very incomplete, increasingly so
further back in time. Despite this, it is often adequate
to illustrate the broader patterns of life's history.
There are also biases in the fossil record: different
environments are more favorable to the preservation of
different types of organism or parts of organisms.
Further, only the parts of organisms that were already
mineralised are usually preserved, such as the shells of
molluscs. Since most animal species are soft-bodied,
they decay before they can become fossilised. As a
result, although there are 30-plus phyla of living
animals, two-thirds have never been found as fossils.
Occasionally, unusual environments may preserve soft
tissues. These lagerstätten allow paleontologists to
examine the internal anatomy of animals that in other
sediments are represented only by shells, spines, claws,
etc. – if they are preserved at all. However, even
lagerstätten present an incomplete picture of life at
the time. The majority of organisms living at the time
are probably not represented because lagerstätten are
restricted to a narrow range of environments, e.g. where
soft-bodied organisms can be preserved very quickly by
events such as mudslides; and the exceptional events
that cause quick burial make it difficult to study the
normal environments of the animals. The sparseness of
the fossil record means that organisms are expected to
exist long before and after they are found in the fossil
record – this is known as the Signor–Lipps effect. |
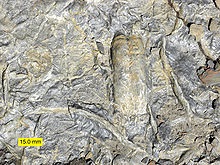 |
Cambrian trace
fossils including Rusophycus, made by a
trilobite. |
Trace fossils
Trace fossils consist mainly of tracks and burrows, but
also include coprolites (fossil feces) and marks left by
feeding. Trace fossils are particularly significant
because they represent a data source that is not limited
to animals with easily fossilised hard parts, and they
reflect organisms' behaviours. Also many traces date
from significantly earlier than the body fossils of
animals that are thought to have been capable of making
them. Whilst exact assignment of trace fossils to their
makers is generally impossible, traces may for example
provide the earliest physical evidence of the appearance
of moderately complex animals (comparable to
earthworms).
Geochemical observations
Geochemical observations may help to deduce the global
level of biological activity at a certain period, or the
affinity of certain fossils. For example, geochemical
features of rocks may reveal when life first arose on
Earth, and may provide evidence of the presence of
eukaryotic cells, the type from which all multicellular
organisms are built. Analyses of carbon isotope ratios
may help to explain major transitions such as the
Permian–Triassic extinction event. |
|
Estimating the
dates of organisms
Paleontology seeks to map out how living things have
changed through time. A substantial hurdle to this aim
is the difficulty of working out how old fossils are.
Beds that preserve fossils typically lack the
radioactive elements needed for radiometric dating. This
technique is our only means of giving rocks greater than
about 50 million years old an absolute age, and can be
accurate to within 0.5% or better. Although radiometric
dating requires very careful laboratory work, its basic
principle is simple: the rates at which various
radioactive elements decay are known, and so the ratio
of the radioactive element to the element into which it
decays shows how long ago the radioactive element was
incorporated into the rock. Radioactive elements are
common only in rocks with a volcanic origin, and so the
only fossil-bearing rocks that can be dated
radiometrically are a few volcanic ash layers.
Consequently, paleontologists must usually rely on
stratigraphy to date fossils. Stratigraphy is the
science of deciphering the "layer-cake" that is the
sedimentary record, and has been compared to a jigsaw
puzzle. Rocks normally form relatively horizontal
layers, with each layer younger than the one underneath
it. If a fossil is found between two layers whose ages
are known, the fossil's age must lie between the two
known ages. Because rock sequences are not continuous,
but may be broken up by faults or periods of erosion, it
is very difficult to match up rock beds that are not
directly next to one another. However, fossils of
species that survived for a relatively short time can be
used to link up isolated rocks: this technique is called
biostratigraphy. For instance, the conodont
Eoplacognathus pseudoplanus has a short range in the
Middle Ordovician period. If rocks of unknown age are
found to have traces of E. pseudoplanus, they must have
a mid-Ordovician age. Such index fossils must be
distinctive, be globally distributed and have a short
time range to be useful. However, misleading results are
produced if the index fossils turn out to have longer
fossil ranges than first thought. Stratigraphy and
biostratigraphy can in general provide only relative
dating (A was before B), which is often sufficient for
studying evolution. However, this is difficult for some
time periods, because of the problems involved in
matching up rocks of the same age across different
continents.
Family-tree relationships may also help to narrow down
the date when lineages first appeared. For instance, if
fossils of B or C date to X million years ago and the
calculated "family tree" says A was an ancestor of B and
C, then A must have evolved more than X million years
ago.
It is also possible to estimate how long ago two living
clades diverged – i.e. approximately how long ago their
last common ancestor must have lived – by assuming that
DNA mutations accumulate at a constant rate. These
"molecular clocks", however, are fallible, and provide
only a very approximate timing: for example, they are
not sufficiently precise and reliable for estimating
when the groups that feature in the Cambrian explosion
first evolved, and estimates produced by different
techniques may vary by a factor of two. |
|
|
|
|
|
|
|
|
|
Search Fun Easy English |
|
|
|
|
|
|
|
About
Contact
Copyright
Resources
Site Map |